Applications of synthetic polymers directed toward living cells
Cells execute remarkable functions using biopolymers synthesized from natural building blocks. Engineering cells to leverage the vast array of synthesizable abiotic polymers could provide enhanced or entirely new cellular functions. Here we discuss the applications of in situ-synthesized abiotic polymers in three distinct domains: intracellular polymerization, cell-surface polymerization and extracellular polymerization. These advances have led to novel applications in various areas, such as cancer therapy, cell imaging, cellular activity manipulation, cell protection and electrode assembly. Examples of these synthetic approaches can be applied across all domains of life, ranging from microbes and cultured mammalian cells to plants and animals. Finally, we discuss challenges and future opportunities in this emerging field, which could enable new synthetic approaches to influence biological processes and functions.
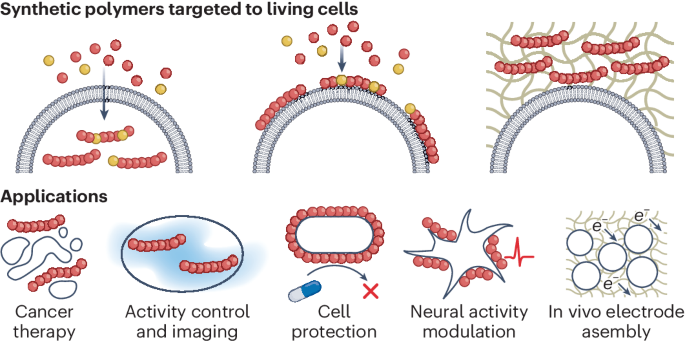
This is a preview of subscription content, access via your institution
Access options
Subscribe to this journal
Receive 12 digital issues and online access to articles
133,45 € per year
only 11,12 € per issue
Buy this article
- Purchase on SpringerLink
- Instant access to full article PDF
Prices may be subject to local taxes which are calculated during checkout
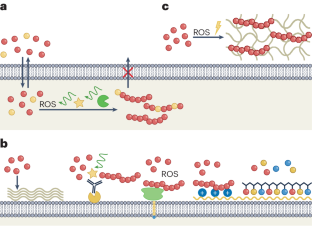
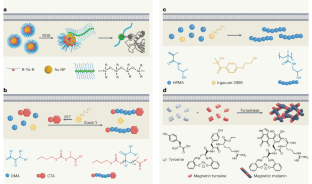
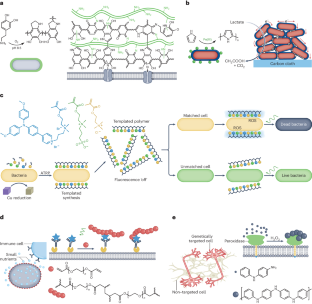
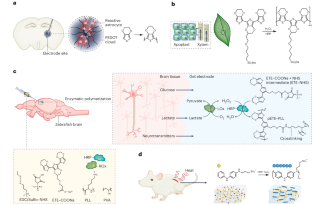
Similar content being viewed by others
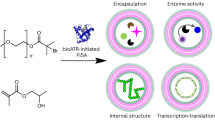
Artificial cell synthesis using biocatalytic polymerization-induced self-assembly
Article Open access 04 December 2023
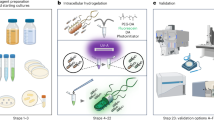
Fabrication of cyborg bacterial cells as living cell–material hybrids using intracellular hydrogelation
Article 22 August 2024
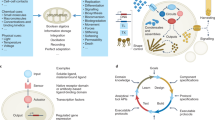
The living interface between synthetic biology and biomaterial design
Article 31 March 2022
References
- Luo, Y. et al. Technology roadmap for flexible sensors. ACS Nano17, 5211–5295 (2023). CASPubMedPubMed CentralGoogle Scholar
- Liu, Y., Feig, V. R. & Bao, Z. Conjugated polymer for implantable electronics toward clinical application. Adv. Healthcare Mater.10, 2001916 (2021). CASGoogle Scholar
- Fang, Y. et al. Dissecting biological and synthetic soft-hard interfaces for tissue-like systems. Chem. Rev.122, 5233–5276 (2022). CASPubMedGoogle Scholar
- Song, E., Li, J., Won, S. M., Bai, W. & Rogers, J. A. Materials for flexible bioelectronic systems as chronic neural interfaces. Nat. Mater.19, 590–603 (2020). CASPubMedGoogle Scholar
- Zeglio, E., Rutz, A. L., Winkler, T. E., Malliaras, G. G. & Herland, A. Conjugated polymers for assessing and controlling biological functions. Adv. Mater.31, 1806712 (2019). Google Scholar
- Tang, T.-C. et al. Materials design by synthetic biology. Nat. Rev. Mater.6, 332–350 (2021). CASGoogle Scholar
- Burgos-Morales, O. et al. Synthetic biology as driver for the biologization of materials sciences. Mater. Today Bio11, 100115 (2021). CASPubMedPubMed CentralGoogle Scholar
- Kightlinger, W., Warfel, K. F., DeLisa, M. P. & Jewett, M. C. Synthetic glycobiology: parts, systems and applications. ACS Synth. Biol.9, 1534–1562 (2020). CASPubMedPubMed CentralGoogle Scholar
- Dai, Y. et al. Oxidative polymerization in living cells. J. Am. Chem. Soc.143, 10709–10717 (2021). CASPubMedGoogle Scholar
- Liu, C. et al. Intracellular hyperbranched polymerization for circumventing cancer drug resistance. ACS Nano17, 11905–11913 (2023). CASPubMedGoogle Scholar
- Lv, N. et al. ROS-initiated in-situ polymerization of diacetylene-containing lipidated peptide amphiphile in living cells. Sci. China Mater.65, 2861–2870 (2022). CASGoogle Scholar
- Kim, S. et al. Intramitochondrial disulfide polymerization controls cancer cell fate. ACS Nano15, 14492–14508 (2021). CASPubMedGoogle Scholar
- Shen, Q. et al. Intracellular radical polymerization of paclitaxel-bearing acrylamide for self-inflicted apoptosis of cancer cells. ACS Mater. Lett.3, 1307–1314 (2021). CASGoogle Scholar
- Zhang, Y. et al. Controlled intracellular polymerization for cancer treatment. JACS Au2, 579–589 (2022). CASPubMedPubMed CentralGoogle Scholar
- Ma, T. et al. Morphological transformation and in situ polymerization of caspase-3 responsive diacetylene-containing lipidated peptide amphiphile for self-amplified cooperative antitumor therapy. Small18, 2204759 (2022). CASGoogle Scholar
- Qi, G. et al. Enzyme-mediated intracellular polymerization of AIEgens for light-up tumor localization and theranostics. Adv. Mater.34, 2106885 (2022). CASGoogle Scholar
- Chen, Y. et al. Nanocompartment-confined polymerization in living systems. Nat. Commun.14, 5229 (2023). CASPubMedPubMed CentralGoogle Scholar
- Geng, J. et al. Radical polymerization inside living cells. Nat. Chem.11, 578–586 (2019). CASPubMedGoogle Scholar
- Liang, G., Ren, H. & Rao, J. A biocompatible condensation reaction for controlled assembly of nanostructures in living cells. Nat. Chem.2, 54–60 (2010). CASPubMedPubMed CentralGoogle Scholar
- Cui, L. et al. Reduction triggered in situ polymerization in living mice. J. Am. Chem. Soc.142, 15575–15584 (2020). CASPubMedPubMed CentralGoogle Scholar
- Li, L.-L. et al. Intracellular construction of topology-controlled polypeptide nanostructures with diverse biological functions. Nat. Commun.8, 1276 (2017). PubMedPubMed CentralGoogle Scholar
- Peng, B., Zhao, X., Yang, M.-S. & Li, L.-L. Intracellular transglutaminase-catalyzed polymerization and assembly for bioimaging of hypoxic neuroblastoma cells. J. Mater. Chem. B7, 5626–5632 (2019). CASPubMedGoogle Scholar
- Nishio, K. et al. Magnetic control of cells by chemical fabrication of melanin. J. Am. Chem. Soc.144, 16720–16725 (2022). CASPubMedGoogle Scholar
- Zhu, M. et al. Tyrosine residues initiated photopolymerization in living organisms. Nat. Commun.14, 3598 (2023). CASPubMedPubMed CentralGoogle Scholar
- Wang, G. et al. Decorating an individual living cell with a shell of controllable thickness by cytocompatible surface initiated graft polymerization. Chem. Commun.54, 4677–4680 (2018). CASGoogle Scholar
- Jiao, C., Zhao, C., Ma, Y. & Yang, W. A versatile strategy to coat individual cell with fully/partially covered shell for preparation of self-propelling living cells. ACS Nano15, 15920–15929 (2021). CASPubMedGoogle Scholar
- Yang, S. H. et al. Mussel-inspired encapsulation and functionalization of individual yeast cells. J. Am. Chem. Soc.133, 2795–2797 (2011). CASPubMedGoogle Scholar
- Pan, C. et al. Polymerization-mediated multifunctionalization of living cells for enhanced cell-based therapy. Adv. Mater.33, 2007379 (2021). CASGoogle Scholar
- Zhong, Y. et al. Site-selected in situ polymerization for living cell surface engineering. Nat. Commun.14, 7285 (2023). CASPubMedPubMed CentralGoogle Scholar
- Song, R.-B. et al. Living and conducting: coating individual bacterial cells with in situ formed polypyrrole. Angew. Chem. Int. Ed.56, 10516–10520 (2017). CASGoogle Scholar
- Ramanavicius, A. et al. Synthesis of polypyrrole within the cell wall of yeast by redox-cycling of [Fe(CN)6] 3− /[Fe(CN)6] 4− . Enzyme Microb. Technol.83, 40–47 (2016). CASPubMedGoogle Scholar
- Magennis, E. P. et al. Bacteria-instructed synthesis of polymers for self-selective microbial binding and labelling. Nat. Mater.13, 748–755 (2014). CASPubMedPubMed CentralGoogle Scholar
- Luo, Y. et al. Synthesis of glycopolymers with specificity for bacterial strains via bacteria-guided polymerization. Chem. Sci.10, 5251–5257 (2019). CASPubMedPubMed CentralGoogle Scholar
- Qi, G. et al. Bacterium-templated polymer for self-selective ablation of multidrug-resistant bacteria. Adv. Funct. Mater.30, 2001338 (2020). CASGoogle Scholar
- Chen, W. et al. Bacteria-driven hypoxia targeting for combined biotherapy and photothermal therapy. ACS Nano12, 5995–6005 (2018). CASPubMedGoogle Scholar
- Chen, W. et al. Combination of bacterial-photothermal therapy with an anti-PD-1 peptide depot for enhanced immunity against advanced cancer. Adv. Funct. Mater.30, 1906623 (2020). CASGoogle Scholar
- Yin, Z. et al. Supramolecular polymerization powered by Escherichia coli: fabricating a near-infrared photothermal antibacterial agent in situ. CCS Chem.4, 3285–3295 (2022). CASGoogle Scholar
- Qi, J. et al. Cyto-friendly polymerization at cell surfaces modulates cell fate by clustering cell-surface receptors. Chem. Sci.11, 4221–4225 (2020). CASPubMedPubMed CentralGoogle Scholar
- Qi, R. et al. In situ synthesis of photoactive polymers on a living cell surface via bio-palladium catalysis for modulating biological functions. Angew. Chem. Int. Ed.60, 5759–5765 (2021). CASGoogle Scholar
- Lilly, J. L., Romero, G., Xu, W., Shin, H. Y. & Berron, B. J. Characterization of molecular transport in ultrathin hydrogel coatings for cellular immunoprotection. Biomacromolecules16, 541–549 (2015). CASPubMedPubMed CentralGoogle Scholar
- Kim, J. Y. et al. Cytocompatible polymer grafting from individual living cells by atom-transfer radical polymerization. Angew. Chem. Int. Ed.55, 15306–15309 (2016). CASGoogle Scholar
- Niu, J. et al. Engineering live cell surfaces with functional polymers via cytocompatible controlled radical polymerization. Nat. Chem.9, 537–545 (2017). CASPubMedGoogle Scholar
- Bennett, M. R., Gurnani, P., Hill, P. J., Alexander, C. & Rawson, F. J. Iron-catalysed radical polymerisation by living bacteria. Angew. Chem. Int. Ed.59, 4750–4755 (2020). CASGoogle Scholar
- Liu, J. et al. Genetically targeted chemical assembly of functional materials in living cells, tissues and animals. Science367, 1372–1376 (2020). CASPubMedPubMed CentralGoogle Scholar
- Zhang, A. et al. Genetically targeted chemical assembly of polymers specifically localized extracellularly to surface membranes of living neurons. Sci. Adv.9, eadi1870 (2023). CASPubMedPubMed CentralGoogle Scholar
- Sessler, C. D. et al. Optogenetic polymerization and assembly of electrically functional polymers for modulation of single-neuron excitability. Sci. Adv.8, eade1136 (2022). CASPubMedPubMed CentralGoogle Scholar
- Zhang, A., Jiang, Y., Loh, K. Y., Bao, Z. & Deisseroth, K. Genetically targeted chemical assembly. Nat. Rev. Bioeng.2, 82–94 (2024). Google Scholar
- Richardson-Burns, S. M. et al. Polymerization of the conducting polymer poly(3,4-ethylenedioxythiophene) (PEDOT) around living neural cells. Biomaterials28, 1539–1552 (2007). CASPubMedGoogle Scholar
- Richardson-Burns, S. M., Hendricks, J. L. & Martin, D. C. Electrochemical polymerization of conducting polymers in living neural tissue. J. Neural Eng.4, L6–L13 (2007). PubMedGoogle Scholar
- Murbach, J. M. et al. In situ electrochemical polymerization of poly(3,4-ethylenedioxythiophene) (PEDOT) for peripheral nerve interfaces. MRS Commun.8, 1043–1049 (2018). CASPubMedPubMed CentralGoogle Scholar
- Ouyang, L., Green, R., Feldman, K. E. & Martin, D. C. in Progress in Brain Research Vol. 194 (eds Schouenborg, J. et al.) 263–271 (Elsevier, 2011).
- Ouyang, L., Shaw, C. L., Kuo, C.-C., Griffin, A. L. & Martin, D. C. In vivo polymerization of poly(3,4-ethylenedioxythiophene) in the living rat hippocampus does not cause a significant loss of performance in a delayed alternation task. J. Neural Eng.11, 026005 (2014). PubMedPubMed CentralGoogle Scholar
- Chen, H.-l, Yang, D., Chen, C.-R., Tian, G.-Z. & Kim, D.-H. In situ polymerization of conducting polymers around living neural cells: cellular effect study. Colloids Surf. B213, 112410 (2022). CASGoogle Scholar
- Zhang, A., Zwang, T. J. & Lieber, C. M. Biochemically functionalized probes for cell-type-specific targeting and recording in the brain. Sci. Adv.9, eadk1050 (2023). CASPubMedPubMed CentralGoogle Scholar
- Bodart, C. et al. Electropolymerized poly(3,4-ethylenedioxythiophene) (PEDOT) coatings for implantable deep-brain-stimulating microelectrodes. ACS Appl. Mater. Interfaces11, 17226–17233 (2019). CASPubMedGoogle Scholar
- Stavrinidou, E. et al. In vivo polymerization and manufacturing of wires and supercapacitors in plants. Proc. Natl Acad. Sci. USA114, 2807–2812 (2017). CASPubMedPubMed CentralGoogle Scholar
- Dufil, G. et al. Enzyme-assisted in vivo polymerisation of conjugated oligomer based conductors. J. Mater. Chem. B8, 4221–4227 (2020). CASPubMedGoogle Scholar
- Parker, D. et al. Biohybrid plants with electronic roots via in vivo polymerization of conjugated oligomers. Mater. Horiz.8, 3295–3305 (2021). CASPubMedGoogle Scholar
- Mantione, D. et al. Thiophene-based trimers for in vivo electronic functionalization of tissues. ACS Appl. Electron. Mater.2, 4065–4071 (2020). CASGoogle Scholar
- Tommasini, G. et al. Seamless integration of bioelectronic interface in an animal model via in vivo polymerization of conjugated oligomers. Bioact. Mater.10, 107–116 (2022). CASPubMedGoogle Scholar
- Strakosas, X. et al. Metabolite-induced in vivo fabrication of substrate-free organic bioelectronics. Science379, 795–802 (2023). CASPubMedGoogle Scholar
- Yokel, R. A. Nanoparticle brain delivery: a guide to verification methods. Nanomedicine15, 409–432 (2020). CASPubMedGoogle Scholar
- Wang, A. et al. H2O2-activated in situ polymerization of aniline derivative in hydrogel for real-time monitoring and inhibition of wound bacterial infection. Biomaterials289, 121798 (2022). CASPubMedGoogle Scholar
- Wang, A. et al. In situ polymerization of aniline derivative in vivo for NIR-II phototheranostics of tumor. ACS Appl. Mater. Interfaces15, 5870–5882 (2023). CASPubMedGoogle Scholar
- Qin, Y. et al. Harnessing oxidative microenvironment for in vivo synthesis of subcellular conductive polymer microvesicles enhances nerve reconstruction. Nano Lett.22, 3825–3831 (2022). CASPubMedGoogle Scholar
- van der Vliet, A. & Janssen-Heininger, Y. M. W. Hydrogen peroxide as a damage signal in tissue injury and inflammation: murderer, mediator or messenger? J. Cell. Biochem.115, 427–435 (2014). PubMedPubMed CentralGoogle Scholar
- Fan, G., Graham, A. J., Kolli, J., Lynd, N. A. & Keitz, B. K. Aerobic radical polymerization mediated by microbial metabolism. Nat. Chem.12, 638–646 (2020). CASPubMedPubMed CentralGoogle Scholar
- Moradali, M. F. & Rehm, B. H. A. Bacterial biopolymers: from pathogenesis to advanced materials. Nat. Rev. Microbiol.18, 195–210 (2020). CASPubMedPubMed CentralGoogle Scholar
- Gilbert, C. & Ellis, T. Biological engineered living materials: growing functional materials with genetically programmable properties. ACS Synth. Biol.8, 1–15 (2019). CASPubMedGoogle Scholar
Acknowledgements
This work was supported by the National Science Foundation Future Manufacturing Program grant (award no. 2037164) and the Keck Foundation. Z.B. is a CZ Biohub-San Francisco Investigator and an Arc Institute Innovation Investigator. A.Z. acknowledges support from the American Heart Association (AHA; award no. 23POST1018301).
Author information
Authors and Affiliations
- Department of Chemical Engineering, Stanford University, Stanford, CA, USA Anqi Zhang, Spencer Zhao, Jonathan Tyson & Zhenan Bao
- Department of Bioengineering, Stanford University, Stanford, CA, USA Anqi Zhang, Jonathan Tyson & Karl Deisseroth
- Department of Chemistry and the Sarafan Chemistry, Engineering & Medicine for Human Health (ChEM-H), Stanford University, Stanford, CA, USA Jonathan Tyson
- Department of Psychiatry and Behavioral Sciences, Stanford University, Stanford, CA, USA Karl Deisseroth
- Howard Hughes Medical Institute, Stanford University, Stanford, CA, USA Karl Deisseroth
- Anqi Zhang